In the modern world of industrial production, waste heat boilers have become indispensable equipment for our operations. We rely on them heavily, and their reliability is absolutely crucial for our entire industrial production process. You see, as the output of our industrial devices fluctuates, the performance of our waste heat boilers becomes even more critical. This is particularly true for the safe operation of our superheaters, which play a pivotal role in the entire setup.
As we continue to push the boundaries and increase the capacity of our waste heat boilers, we’ve noticed that certain parameters are constantly on the rise. Unfortunately, so are incidents of superheater pipe bursts. Even when we’ve adjusted the superheater outlet design parameters by reducing them by 5 to 15 degrees Celsius, these pipe burst occurrences stubbornly persist.
While there’s been extensive analysis in various literature on the pipe burst phenomenon in power station boiler superheaters, it’s become clear that the main culprit is local over-temperature. However, in the realm of high-parameter waste heat boilers, it’s not just local over-temperature causing these pipe bursts; there’s also the looming threat of corrosion eating away at our pipes.
To unravel the mystery of why large-scale tube bursts were occurring in superheaters in such a short span, we embarked on a comprehensive investigation. We hit the field, conducted surveys, and collected samples from the furnace tubes. We delved into the material composition and delved deep into metallurgical aspects to piece together the puzzle.
In this paper, we’ll take a close look at a specific incident where the secondary superheater pipes in a high-temperature, high-pressure waste heat boiler burst. We’ll dissect the root causes and, importantly, present corrective measures to ensure smoother operations moving forward.
Superheater Pipe Burst: A Troubling Situation
1.1 The Unfortunate Superheater Tube Bursts
Let me share a scenario with you: at a user’s waste heat boiler, after an overhaul involving the replacement of heating surfaces, everything seemed fine for a few months. However, it didn’t take long for trouble to arise. Two superheater desuperheater tubes at the back end of the boiler suddenly burst. After diligent repair and resumption of operations, disaster struck again – this time, superheater pipes kept bursting, all in a remarkably similar location.
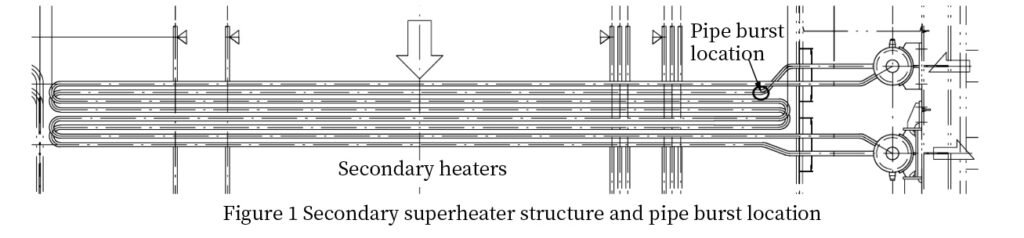
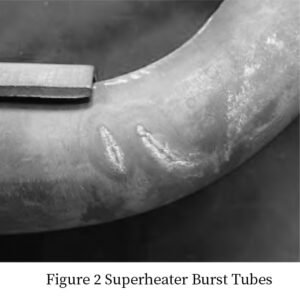
Upon a closer look during an internal boiler inspection after shutting down the furnace, it became apparent that the bursts were concentrated near the collector box elbow bend of the secondary superheater pipes. The cracks radiated outwards, numbering around 5 to 10 distinct ones. (Refer to Figures 1 and 2 for a visual representation.) These superheater tubes were made of 07Cr19Ni11Ti material, with dimensions of ϕ38 × 4.
1.2 Digging into the Boiler’s Operations
When the second round of pipe bursts occurred and we had to halt operations again, we decided to conduct a thorough investigation on-site. Our goal? To understand the factors responsible for these frustrating bursts. Here’s what we discovered:
(1) Water Quality Matters: Our boiler water quality sampling and analysis unveiled a concerning revelation. The water samples contained high levels of halogen elements, particularly F ions and Cl ions, reaching an astonishing 100 mg/L.
(2) Superheater Spray Water Investigation: Our scrutiny extended to the superheater spray water and its quality.
Desuperheater Troubles: The superheater water spray desuperheater used a variable throttle orifice design, with a plunger that automatically adjusted its opening based on steam flow. This system is versatile, accommodating a wide range of operating conditions. Unfortunately, during our inspection, we discovered that the plunger’s spring had broken and there were leaks both internally and externally (see Figures 4 and 5). This malfunction disrupted the desuperheater’s atomization, allowing water to mix with the steam entering the superheater. Consequently, there were sharp temperature fluctuations in the main steam, ranging from 415 to 485°C, causing significant temperature variations in the superheater tube walls.
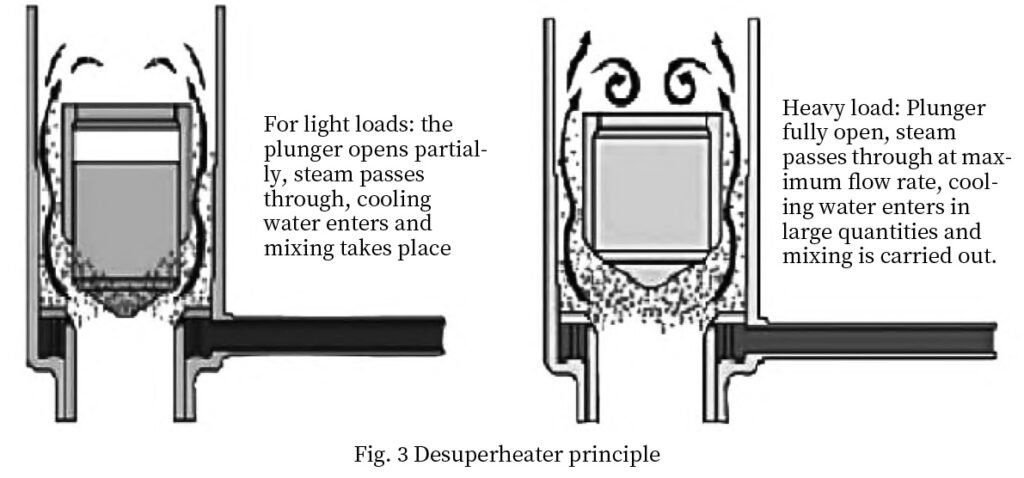
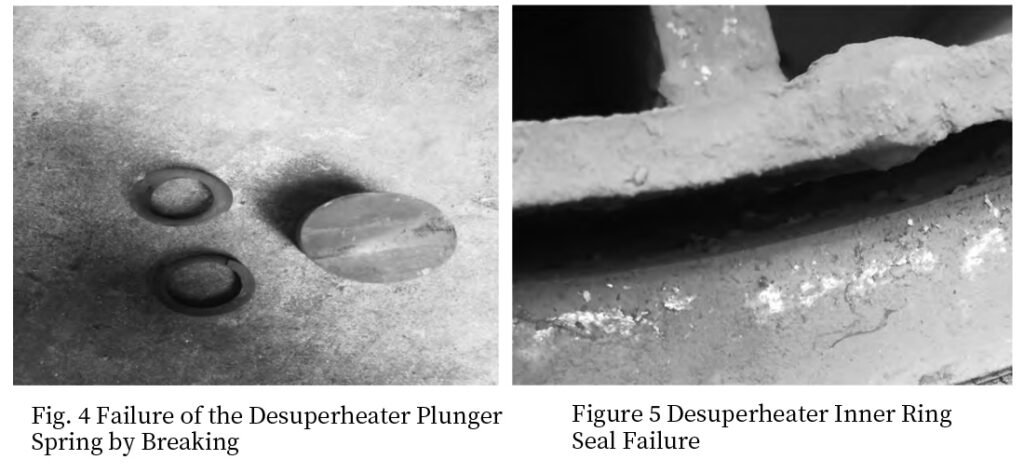
(3) Lack of Automatic Adjustment: To make matters worse, the boiler’s three-stroke automatic adjustment system wasn’t functioning correctly. This resulted in noticeable temperature regulation delays, which manifested as steam over-temperature and superheater tube overheating. The failed water spray atomization further exacerbated the situation, as water infiltrated the superheater tubes, intensifying the thermal stress they endured.
Superheater Tube Testing and Analysis: Uncovering the Story
2.1 Diving into Chemical Composition
When it comes to understanding the integrity of our superheater tubes, we start with a chemical composition analysis. We followed the test standard GB/T 11170-2008, “Stainless Steel Multi-Element Content Determination by Spark Discharge Atomic Emission Spectrometry (Conventional Method),” and consulted GB/T 5310-2017 standards. The results revealed that the chemical composition of the superheater tube material fell comfortably within the standard deviation range (+0.005%). In other words, the tube samples checked out and met the standard requirements for 07Cr19Ni11Ti steel. You can refer to Table 1 for the detailed breakdown of the pipe’s chemical composition analysis results.
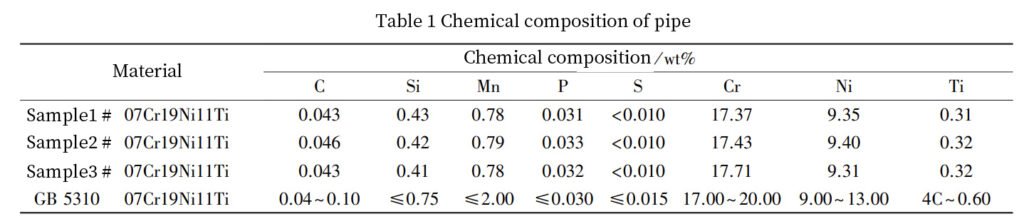
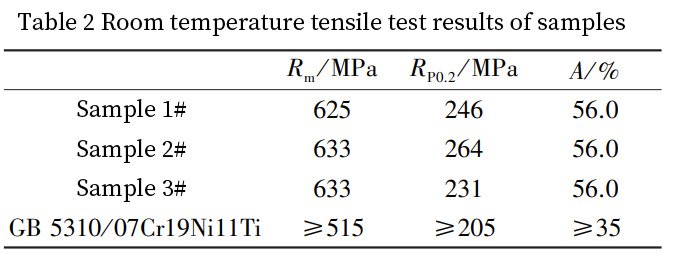
2.2 Room Temperature Tensile Test
We didn’t stop at the chemical analysis; we also conducted room temperature tensile tests. Using the sampling method laid out in GB/T 228.1-2010, “Metal Materials Tensile Test Part 1: Room Temperature Test Method,” we sampled straight sections of the superheater pipe for testing, employing arc tensile specimens. The results came back positive, showing that the pipe’s room temperature tensile properties met the GB/T 5310-2017 standards for 07Cr19Ni11Ti steel.
2.3 Getting Hard with Hardness Testing
Our journey into assessing these superheater tubes also involved hardness testing, following the GB/T 4340.1-2009 standard, “Metal Materials Vickers Hardness Test Part 1: Test Methods.” The results indicated that the hardness values of the pipe section’s metallographic samples were in proximity to the 07Cr19Ni11Ti steel hardness range of 150 to 200 HV. However, there was a slight deviation on the lower limit for the straight pipe and elbow samples.
2.4 Peering into the Microstructure
To gain a deeper insight, we ventured into metallographic testing. We intercepted transverse metallographic specimens from the straight pipe section and obtained longitudinal or transverse metallographic specimens from portions of the bend section where cracks were present. Here’s what we found:
(1) Straight Section Microstructure: The microstructure consisted of twin austenite with a grain size of about 4 to 6 levels. There were no noticeable anomalies in the organization, although the inner wall exhibited slight micro-pit or micro-crack characteristics.
(2) Elbow Microstructure: Similar to the straight section, the elbow’s microstructure showcased twin austenite with evident twin deformation (more pronounced at the 10.5° elbow sampling point). Grain size was around 4 to 6 with deformation characteristics, and the inner wall displayed slight micro-pit or micro-crack features.
(3) Crack Characteristics: The cracks at the elbow’s neutral surface exhibited a typical crystallographic cracking pattern. The section showed an abundance of corrosion products and secondary cracks. These secondary cracks expanded from the inner wall side to the outer wall side, with a noticeable step-like source area on the inner wall side. Chemical analysis revealed higher F and Cl elements on the inner wall side, while the outer wall side had more Cl elements and a smaller amount of F. Both inner and outer walls showed the presence of S and N elements, along with Ca, Al, Si, and other elements.
2.5 Uncovering Residual Stress
Lastly, we delved into the world of residual stress testing, as per SAE J784A:1971 and ASTM E915:2010 standards. We sampled the elbow position of the pipe, examining the outer wall side, inner wall side, and the neutral surface for residual stress. Our findings were intriguing: the elbow section displayed high hardness, grain deformation, and twinning. The intergranular stress corrosion on the inner wall side, mainly attributed to F elements, was caused by halide elements, particularly NaF, which could hydrolyze to produce HF. This corrosion was closely linked to the corrosion scale composed mainly of S and Cl elements, alongside elements such as Na and Si. This hydrolysis process led to HF production, as shown in the equation: HF: NaF + H2O → HF + NaOH. Interestingly, the outer wall side showed no signs of Cl, S, or other corrosion-related elements. Ultimately, it was the intergranular stress corrosion defects and crack propagation through the pipe wall, originating from the inner wall, that culminated in pipe bursts and leakage.
Understanding the Causes of Pipe Bursts and Implementing Preventive Measures
(1) Root Causes Unveiled From our meticulous analysis, it’s clear that the primary culprit behind the superheater pipe bursts can be traced back to two main factors:
Desuperheater Malfunction: Prolonged operation of the water jet desuperheater took a toll, leading to the fracture of the desuperheater plunger spring. This malfunction disrupted the atomization process, allowing steam to mix with water and infiltrate the superheater.
Water Quality Issues: Compounding the problem, subpar water treatment resulted in higher concentrations of halogenated elements in the water. This concentration, especially at the elbow of the heat exchanger tube, fostered conditions for droplet accumulation. This, in turn, triggered intergranular stress corrosion on the inner wall side, ultimately leading to cracks.
(2) Preventive and Control Measures:
To address these issues and prevent future superheater pipe bursts, we recommend the following proactive steps:
Improved Water Quality Management: Strengthen the management of waste heat boiler water quality. Specifically, take measures to reduce the Cl ion content in the water. This will mitigate the risk associated with halogenated elements in the water supply.
Regular Inspection and Maintenance: During annual boiler inspections, ensure a thorough check of the internal components of the desuperheater. Replace the plunger spring regularly to maintain its reliability and functionality.
Desuperheating System Enhancement: Consider modifying the desuperheating water feedwater regulating valve to minimize any delays in its operation. This modification will help ensure the desuperheater responds promptly and accurately to control steam temperature.
Enhanced Operation Management: Strengthen overall boiler operation management, with a particular focus on the daily observation and maintenance of the desuperheater. Regular monitoring and timely maintenance will help identify and address potential issues before they escalate. By implementing these preventive and control measures, we aim to enhance the reliability and safety of our waste heat boiler, minimizing the risk of superheater pipe bursts and ensuring smooth and trouble-free industrial production.

In Conclusion: Safeguarding Waste Heat Boiler Operations
In our investigation, we’ve pinpointed the primary causes behind the secondary superheater tube bursts in the user’s waste heat boiler. The main culprits are:
Desuperheater Atomization Failure: The extended operation of the desuperheater led to a plunger spring fracture, disrupting the water jet atomization process. This failure allowed water to mix with steam, infiltrating the superheater.
Water Quality Concerns: Insufficient water treatment resulted in higher concentrations of halogenated elements in the water. This accumulation, particularly at the elbow of the heat exchanger tube, triggered intergranular stress corrosion and ultimately led to tube bursts.
Additionally, significant temperature fluctuations exacerbated the situation, increasing thermal stress on the tubes and culminating in bursts at the elbow.
To ensure the safe operation of high-parameter waste heat boilers with stainless steel superheaters, we recommend the following key measures:
Water Quality Control: Implement strategies to reduce the Cl ion content in the feedwater, addressing the issue of halogenated elements in the water supply.
Regular Maintenance: During annual boiler inspections, prioritize the thorough examination of the desuperheater components. Regularly replace the plunger spring to maintain its reliability and functionality.
Desuperheater Enhancements: Consider modifications to the desuperheating system, such as improving the water feedwater regulating valve to reduce response delays, ensuring the system operates effectively.
Enhanced Operation Management: Strengthen overall boiler operation management, with a specific focus on daily observation and maintenance of the desuperheater. Timely monitoring and maintenance can help detect and address potential issues before they escalate.
By adhering to these preventive and control measures, we can safeguard the safe and reliable operation of waste heat boilers, particularly those with high parameters and stainless steel superheaters. This proactive approach will help minimize the risk of superheater tube bursts and contribute to the uninterrupted and secure operation of industrial processes.
DHB Boiler
Discover The Superior Quality And Cutting-Edge Technology Of DHB Boilers. Explore Our Range Of Biomass Boilers, Waste Heat Boilers, And More. Take Your Industrial Operations To New Heights With DHB Boiler.