Unraveling the Enigma: Understanding High-Temperature Corrosion on Boiler Heating Surface
With a focus on advocating green practices, environmental protection, and adopting a low-carbon lifestyle, waste incineration power generation has gradually become the mainstream method for domestic waste treatment, characterized by its attributes of “harmlessness, resourcefulness, and reduction.” As China’s domestic waste production continues to escalate, a substantial number of high-parameter, large-capacity waste incineration boilers have been deployed. The safe operation of these boilers is paramount as it directly impacts the safety of people’s lives and property and significantly influences the progress of environmental protection measures for urban domestic waste treatment.
Moreover, in tandem with the improvement of people’s living standards, the average calorific value of domestic garbage is also on the rise, surpassing the initial design considerations of incineration boilers.
Additionally, the irregular shape of the waste, coupled with variations in quality across seasons and regions, results in a significant disparity in the corresponding calorific values. This leads to fluctuations in the flue gas temperature and composition during the waste incineration process. In Figure 1, the structure of a typical waste incineration boiler is depicted, wherein household garbage undergoes combustion after passing through the grate and transferring to the bottom of the furnace. Subsequently, the high-temperature flue gas interacts with the furnace water-cooled wall and horizontal flue superheater, along with other heated surfaces. In this complex setting, characterized by high temperature, high pressure, vibrations, corrosion, and a gas-solid-liquid multiphase flow environment, the heating surface tubes are susceptible to high-temperature corrosion. This corrosion leads to the thinning of the tube wall and may even result in burst tubes, posing a continuous threat.
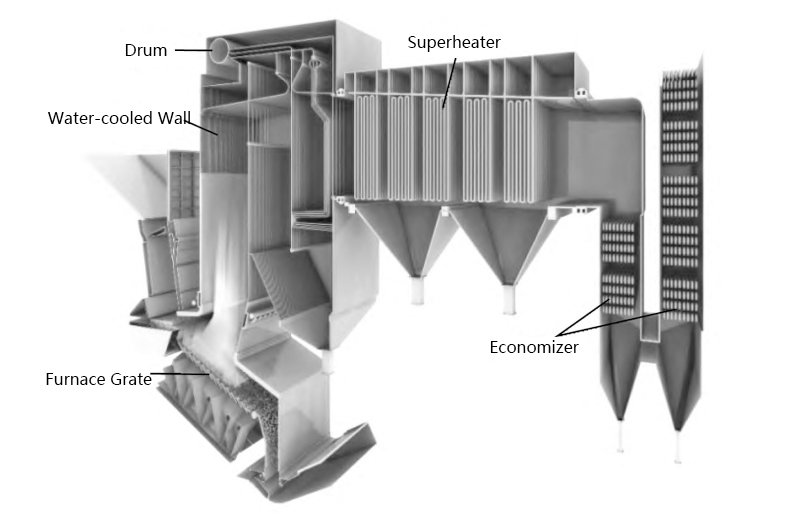
Therefore, extensive research into the mechanisms of high-temperature corrosion on the waste incineration boiler heating surface tubes is imperative. This research aims to enhance preventive measures and establish scientific and effective procedures for preventive inspections and safety evaluations of the waste incineration boiler’s heating surface.
Waste incineration boiler heating surface high-temperature corrosion failure mechanism
High-temperature corrosion refers to the irreversible physical or chemical reaction and degradation process of metal materials in environmental media at elevated temperatures. Essentially, it involves the oxidation process of metal, wherein the metal loses electrons, and the corrosion media gains electrons. The term “high temperature” typically denotes a working environment temperature that reaches 0.3 to 0.4 times the melting point of the metal, which, in the case of the commonly used steel in boilers, is generally 500 to 600°C or higher, qualifying it as a high-temperature corrosion environment.
Household garbage exhibits the characteristics of complex component compositions, and its combustion generates a significant amount of corrosive gases, salts, fly ash particles, and involves gas-solid-liquid multi-reaction mixing development. These substances transfer within porous media through various interactive processes, including homogeneous and heterogeneous interactions, grain boundary processes, electrochemical processes, and stress evolution, among others. Consequently, multiple factors contribute to high-temperature corrosion on waste incineration boiler heating surfaces. Depending on the forms of corrosive media, high-temperature corrosion on the boiler heating surface can be broadly categorized into three types:
1.1 High-temperature gaseous corrosion
High-temperature gaseous corrosion, also known as dry corrosion, becomes prominent when domestic garbage contains a significant proportion of plastics and rubber products. The combustion of these substances generates corrosive gases such as HCl and Cl2, which come into contact with the metal layer and oxidized layer of the boiler heating surface pipe. The reaction between these gases and the metal leads to the formation of metal chlorides with low melting points and high vapor pressures, making them highly volatile. As a result, the boiler heating surface pipe reacts readily with chlorine-containing gas, leading to the gradual corrosion of the original metal material in the form of metal chloride, thereby resulting in high-temperature corrosion.
1.2 High-temperature liquid corrosion
High-temperature liquid corrosion, also known as thermal corrosion, occurs due to the presence of liquid molten salts, low melting point metal oxides, and liquid metals (e.g., Pb, Sn) in the high-temperature flue gas produced during the incineration process. These liquid substances act as corrosion media and cause early degradation of the metal properties of the boiler heating surface due to severe erosion. Over time, the metal surface in the high-temperature furnace and horizontal flue tubes accumulates combustion dust, which contains metal oxides, alkali metals, and other heavy metal chlorides and sulfates. Among them, PbCl2, ZnCl2, and SnCl2 are low-melting-point chlorides, while other chlorides like NaCl, KCI, and FeCl3 can form low-melting-point eutectic mixtures when combined with other substances. These liquid-phase media can dissolve the oxide film, considerably increasing the corrosion rate of the metal materials of the boiler heating surface’s high-temperature components. As the corrosion progresses, the heating surface tube develops pits, and eventually, the corrosion-induced thinning may lead to leakage and pipe bursts, as depicted in Figure 2.

1.3 High-temperature solid state corrosion
High-temperature solid-state corrosion, also referred to as abrasion or erosion, primarily occurs due to the impact of fly ash particles generated during combustion. These particles scour and locally wear down the external surface of the boiler heating surface, particularly in regions with higher flue gas and fly ash concentrations. The kinetic energy of fly ash particles in the high-temperature combustion environment causes them to impact the wall of the heating surface tube, leading to the removal of a small amount of metal debris. Over time, this accumulation results in visible wear or erosion. Figure 3 illustrates an example of leakage in the heating surface tube caused by flue gas erosion in a waste incineration boiler.

High-temperature corrosion countermeasures for the boiler heating surface
To effectively address high-temperature corrosion on the heating surface of waste incineration boilers and ensure their efficient and stable operation, it is crucial to implement targeted countermeasures, conduct preventive boiler inspections, and carry out safety evaluations. Timely detection and elimination of equipment defects are essential to maintain a safe, environmentally friendly, and economically viable operation.
2.1 Adjustment of the operation process
The improper control of the operation process during refuse incineration boiler operation can lead to fluctuations in flue gas temperature, resulting in a doubled corrosion rate on the boiler heating surface. To mitigate this, the operating unit should regularly analyze the calorific value of the waste entering the furnace and, if necessary, ensure thorough mixing to stabilize the furnace temperature as much as possible. Controlling the outlet temperature and flow rate of the flue gas in the furnace chamber can be achieved by adjusting the primary and secondary air volume, ratio, and air temperature. Utilizing feedback adjustments based on data from measuring points in the furnace and employing simulation models using relevant finite element simulation software (as depicted in Figure 4), numerical simulations can be carried out to analyze the temperature of each area in the furnace, flue gas flow rate, and the concentration of each component under different working conditions. This information can be used to optimize the primary and secondary air ratio, ensuring the heating surface structure and flue gas emission meet usage requirements and standards.

To address the challenge of significant changes in waste calorific value, promoting waste classification or coarse screening upon entry into the plant can stabilize the furnace temperature and prevent overheating of the boiler heating surface. Regular cleaning of the heating surface is recommended to prevent excessive ash accumulation, thereby enhancing thermal efficiency and reducing metal corrosion on the boiler heating surface. Additionally, adding fuel additives in the waste incineration boiler can improve the physical and chemical properties of ash or generate metal salts that are less prone to corrosion at high temperatures, effectively inhibiting corrosion occurrence.
2.2 Optimization of the heating surface structure
Early designs of waste incineration boilers did not account for the substantial increase in domestic garbage calorific value. Some waste incineration power plants, aiming to increase garbage treatment capacity and achieve short-term economic gains, led to overloaded boiler operations and evident overheating of the heating surface tubes. To tackle this issue, modifications can be made to the boiler’s heating surface structure, such as increasing the front evaporator and transforming the superheater countercurrent. These modifications can help avoid high-temperature corrosion of the superheater due to the high flue gas temperature. Figure 5 illustrates a schematic diagram of medium-temperature superheater countercurrent transformation in a waste incineration power plant in Shanghai. Calculating the import and export wall temperature under working conditions reveals that after the transformation, the high-temperature flue gas side wall temperature at point A significantly reduces, while the low-temperature flue gas side wall temperature at point B increases but remains lower than the wall temperature at point A before the transformation. This effective reduction in wall temperature at point A helps prevent high-temperature corrosion on the boiler heating surface.

2.3 Operation monitoring for the boiler heating surface
High-temperature corrosion on the boiler heating surface can be effectively evaluated through the implementation of furnace shutdowns for inspection and operation monitoring. Currently, furnace metal supervision and inspection are prioritized, but this approach lacks preventive capabilities. To address this, both domestic and international on-line monitoring techniques for high-temperature corrosion have been developed, including:
- Utilizing spraying technology to integrate thin copper-coated optical sensing fibers into the coating to monitor the boiler heating surface’s heat transfer surface.
- Employing a new type of high-temperature electrochemical sensors, coupled with wireless communication and energy-harvesting thermoelectric generators (TEG), to monitor high-temperature corrosion.
- Implementing three-electrode electrochemical corrosion rate (ECR) sensors to measure high-temperature corrosion rate.
Additionally, studying the correlation between the concentration of SO2, H2S, and CO in the flue gas composition in the combustion area of the boiler and the high-temperature corrosion rate can establish a high-temperature corrosion model. By monitoring the concentration of flue gas composition at the water-cooled wall in real-time applications, the actual high-temperature corrosion rate can be obtained, providing valuable insights for optimizing boiler operations. Employing effective operation monitoring means allows real-time tracking of high-temperature corrosion on the heating surface, enabling prompt countermeasures to reduce the risk of accidental pipe bursts.
2.4 Surface protection against corrosion for the boiler heating surface
To reduce the corrosion caused by high-temperature corrosive environmental media on the surface of the boiler heating surface tubes, surface protection and anti-corrosion measures can be implemented. Surface anti-corrosion involves adding a layer of nickel-based anti-corrosion material to the surface of the heating surface. This can be achieved through various processing techniques, such as:
(1) Cold spraying, where high-pressure air gun spraying forms a composite crystalline film, including nano-ceramics, on the heating surface. However, due to uncontrollable finished product quality, this technique is less used in waste incinerators.
(2) Thermal spraying, commonly used with flame spraying, exhibits strong material adaptability. However, the spraying layer’s low bonding force (<100 MPa) and high porosity (>1%) limit its ideal application on waste incineration boiler heating surfaces.
(3) Surfacing, which involves using conventional manual welding or other welding methods to melt alloy material wire and coat it on the heating surface matrix. Surfacing technology is mature, reliable, and more widely used, but it comes with higher costs, lower efficiency, and potential impacts on the base material’s mechanical and performance properties.
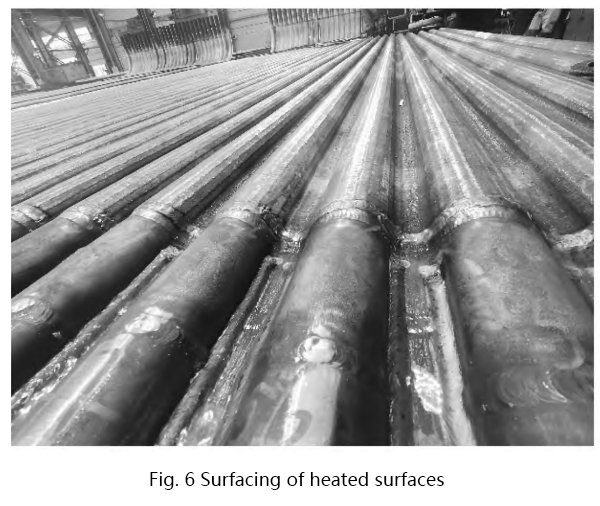
(4) Laser cladding, where a high-energy density laser beam melts the sprayed metal anti-corrosion powder and the heated surface material simultaneously. The resulting cladding layer has an extremely low dilution rate and metallurgical bonding between the anti-corrosion material and the heated surface pipe. Laser cladding shows promise for resisting high-temperature corrosion and wear resistance, making it a focus of both domestic and international research and promotion.
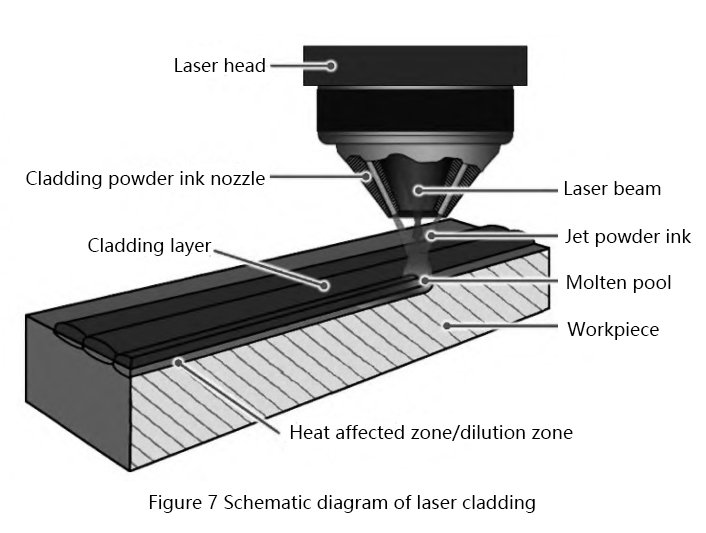
(5) Micro-melt welding, similar to laser cladding, involves initially thermal spraying corrosion-resistant alloy powder on the heating surface, then using high-frequency electric heating or flame heating to melt the cladding layer and create a solid solution diffusion layer. This leads to a combination of the alloy powder and the substrate.
In summary, cladding technology is the most widely used, but it has drawbacks such as higher cost, lower efficiency, and a higher dilution rate. It is generally suitable for medium-temperature and medium-pressure waste heat boilers. Laser cladding technology is less efficient, and its domestic development has not yet matured, but it excels in resisting high-temperature corrosion and wear resistance, making it a hot topic for research and promotion. Micro-welding technology has little thermal impact on the substrate, lower cost, and is suitable for large-scale configuration and use in waste incineration boilers.
Waste incineration boiler high-temperature corrosion prevention and safety evaluation for the boiler heating surface
To effectively curb high-temperature corrosion and ensure the long-term safe and efficient operation of waste incineration boilers, it is essential to implement protective measures on the heating surface while addressing the source of high-temperature corrosion. A comprehensive analysis of the high-temperature corrosion mechanism in different regions of the heating surface is required to identify key factors contributing to the corrosion. This analysis guides targeted adjustments to the operational process. The following steps can be taken:
Firstly, on-site sampling of actual waste incineration boiler slag and corrosion products can be conducted for microscopic analysis. Utilizing techniques such as X-ray diffraction, scanning electron microscopy, energy spectrum analysis, and X-ray fluorescence spectrometry, corrosion products in different areas can be analyzed to differentiate and study the corrosion mechanism under various conditions.
Secondly, conducting experiments on typical heat-resistant steels and nickel-based alloys present on the high-temperature heating surface in a laboratory setup simulating the real flue gas atmosphere. Analyzing the phase stability and microstructure of the corrosion products helps reveal the high-temperature corrosion reaction mechanism of the multifunctional system in the presence of chlorine and alkali metals in the flue gas atmosphere.
Furthermore, utilizing a computer finite element simulation system enables the numerical simulation of gas-solid-liquid flow on the heating surface. This helps establish a material transport model between the combustion environment and the material interface, enabling the prediction of the corrosion rate. These predictions provide a basis for containing high-temperature corrosion of the boiler heating surface tube.
For promoting the long-term safe and efficient operation of waste incineration boilers, a reasonable safety evaluation is of great significance. This involves:
- Qualitative and quantitative analysis of the technical level of the operation and inspection personnel, their sense of responsibility, and other factors related to the operation of the waste incineration boiler.
- Comprehensive analysis of the incineration boiler’s operating conditions, structural characteristics, fuel components, combustion products, operating systems, maintenance programs, and key environmental factors that may impact equipment stability.
- Measurement of the impacts of failure of pressurized parts and establishing a fuzzy evaluation mathematical model for safety evaluation of waste incineration boilers.
- Combining the operation process, component structure, safety accessories, environment, personnel, and management of the waste incineration boiler to give a total score and evaluation level through the fuzzy evaluation mathematical model.
- Providing qualitative suggestions to address the identified issues.
In conclusion, understanding the mechanism of high-temperature corrosion and making adjustments to the operation and maintenance technologies can effectively reduce the impact of high-temperature corrosion on the heating surface of waste incineration boilers. Implementing a scientific and effective safety evaluation of pressurized parts further enhances the safe operation of waste incineration boilers.
[Source] Chenle, Industry Boiler:1004-8774( 2023) 03-0024-05
DHB Boiler
Discover The Superior Quality And Cutting-Edge Technology Of DHB Boilers. Explore Our Range Of Biomass Boilers, Waste Heat Boilers, And More. Take Your Industrial Operations To New Heights With DHB Boiler.