S30432 steel, enriched with a distinctive blend of elements including 0.1% carbon, 18% chromium, 9% nickel, 3% copper, and niobium (Nb), stands as an upgraded iteration of TP304 steel. The infusion of copper (Cu) and niobium (Nb) at concentrations of 3% and 0.5% respectively, initiates a remarkable transformation in the steel’s composition. This alteration leads to the emergence of copper-rich phases, carbides, and nitrides containing niobium – a phenomenon that significantly enhances the steel’s resistance to high-temperature creep. As a result, S30432 steel becomes a prime candidate for demanding applications, offering exceptional oxidation resistance. It becomes an optimal choice, especially in the realm of supercritical and ultra-supercritical steam superheaters, particularly in the final stages of superheating and high-temperature re-heating.
While S30432 steel shines in high-temperature environments within thermal power units, there is still ample room for exploration and research in this field. Present investigations tend to focus on specific issues such as pipe bursts or leaks occurring in welded joints, elbows, or straight pipe sections. These problems can often be traced back to factors like inherent manufacturing defects, lapses in process execution, installation challenges, and the consequences of operating at elevated temperatures. However, the comprehensive analysis of the underlying precipitation phases in these scenarios remains a relatively uncharted territory.
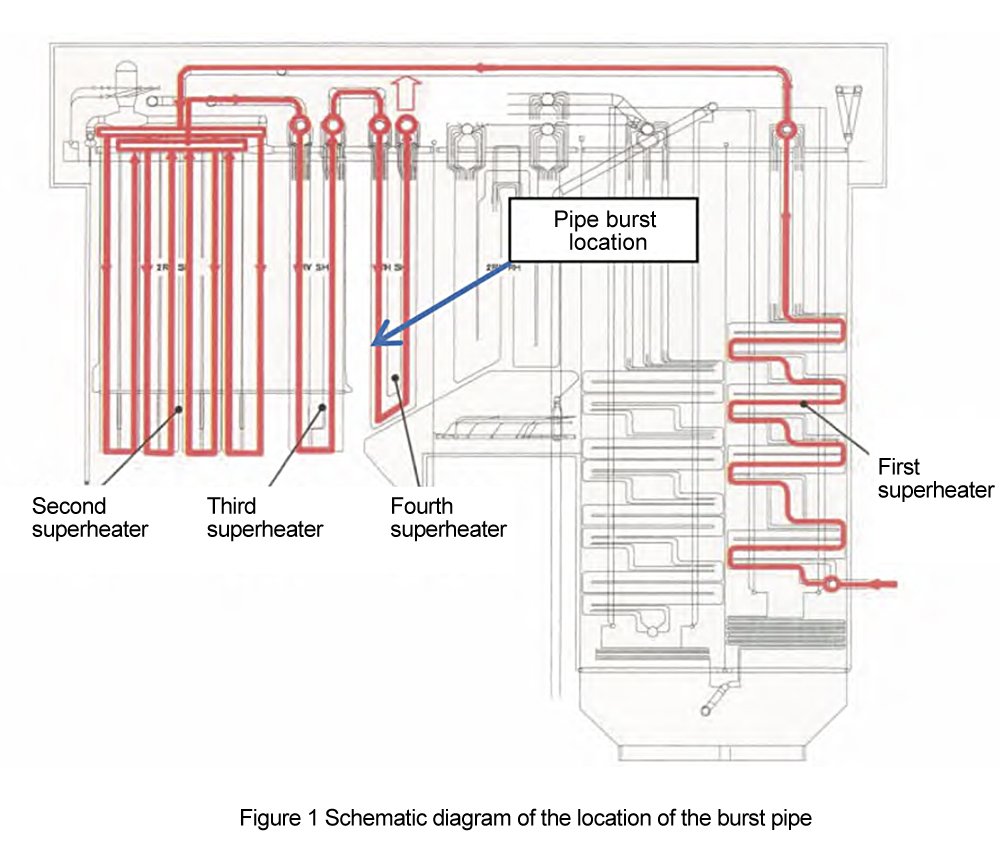
To paint a clearer picture, let’s take a closer look at a real-world scenario. Imagine a superheater system with a four-stage arrangement, featuring a total of 35 panels of serpentine tubes for the screen superheater. Each panel comprises 19 tubes, and these tubes are made from S30432 steel, as well as S31042 steel. The final superheated steam from this system reaches an impressive 26.15MPa in pressure and a scorching 605°C in temperature. The reheated steam, which follows, has outlet pressures of 4.98MPa and 4.78MPa, coupled with a temperature of 603°C.
Within this complex system, an issue arises. The 14th pipe on the outlet collector side of the screen superheater experiences a burst. This breach occurs at the elbow’s outlet section, around 6 meters to the left and right of the collector, and approximately 10 meters below the ceiling. The entire row of pipes sits at a height of about 16 meters. These pipes, constructed from S30432 steel, have a specification of φ51mm in outer diameter and a 9.5mm wall thickness. By the time of this incident, the boiler has seen around 60,000 hours of cumulative operation. The exact location of the burst pipe is depicted in Figure 1.
In a nutshell, S30432 steel is designed to handle high-temperature and high-stress conditions, and although it’s widely used in demanding applications, there’s still much to learn about its behavior and potential challenges in real-world settings. The situation we’ve just described offers a glimpse into the intricacies of dealing with unexpected incidents in these critical systems.
Physical and chemical tests of Superheater
In our examination of the burst pipe from the screen superheater, we conducted a range of physical and chemical tests to gain a deeper understanding of what had transpired.
1.1Macroscopic Observation:
- Figure 2 illustrates the macroscopic morphology of the burst pipe. Upon close inspection, it becomes evident that the pipe has undergone substantial plastic deformation. It bent approximately 45 degrees at the point of rupture, resulting in longitudinal cracks that expanded along the pipeline, forming a small, flare-shaped burst.
- The total length of the burst measured 530 mm, with the widest point of the rupture mouth spanning about 85 mm, and the maximum circumference of the ring being 240 mm.
- Near the mouth of the burst, there were no apparent signs of thickening. Instead, we noticed several longitudinal cracks running parallel to the mouth’s direction.
- The most significant burst had a slightly thinned wall, characterized by blunt edges and brittle cracking. The inner surface of the burst was smooth, while the outer surface showed severe scouring with longitudinal cracks.
- At the point where the pipe wall burst, we observed slight thinning, with similarly blunt edges and brittle fracture characteristics. Once again, the inner surface was smooth, but the outer surface exhibited corrugations from serious scouring. Notably, there was no obvious presence of oxide skin at the largest opening.
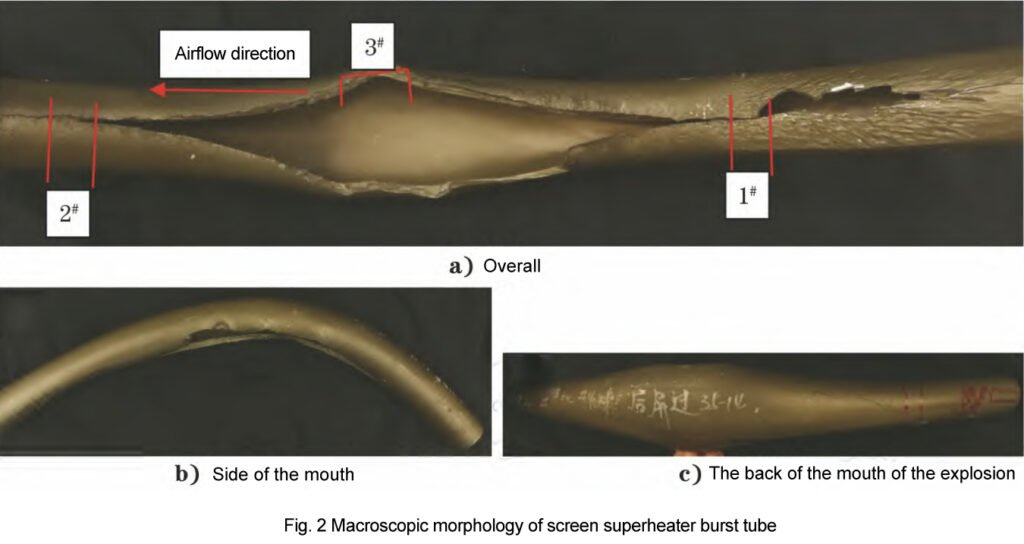
The location where we obtained samples from the burst pipe is depicted in Figure 2a). The direction of steam flow is indicated by the arrow in Figure 2a). We extracted three samples for closer analysis:
- At the mouth of the largest burst (3# sample), which we used for cross-section morphology examination with a scanning electron microscope (SEM).
- At the end of the burst (1# sample), in the direction of steam flow.
- Also at the end of the burst, but perpendicular to the steam flow (2# sample).
1.2 Chemical Composition Analysis of Superheater :
For chemical composition analysis, we utilized a direct-reading spectrometer on the 1# sample. At intervals of 120 degrees, we took measurements at three different points. The results of this analysis are summarized in Table 1.
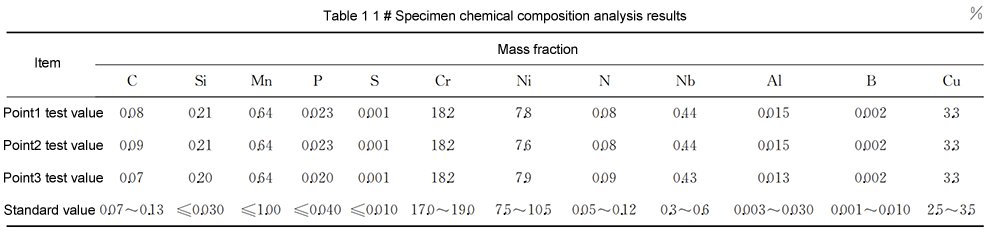
The data from Table 1 indicates that the composition of the sample aligns with the requirements set forth in ASMESA-213-2019, which pertains to “Seamless ferritic and austenitic alloy steel tubes for boilers, superheaters, and heat exchangers.”
1.3 Hardness Test of Superheater :
We conducted a Vickers hardness test on the annular 1# specimen on the inlet side and the annular 2# specimen on the outlet side of the bursting port. The test involved applying a load of 1.961N for a duration of 15 seconds. We also performed the test on both the precipitation phase and non-precipitation areas, employing a load of 0.09807N for 15 seconds.
The results of these hardness tests are presented in Table 2.
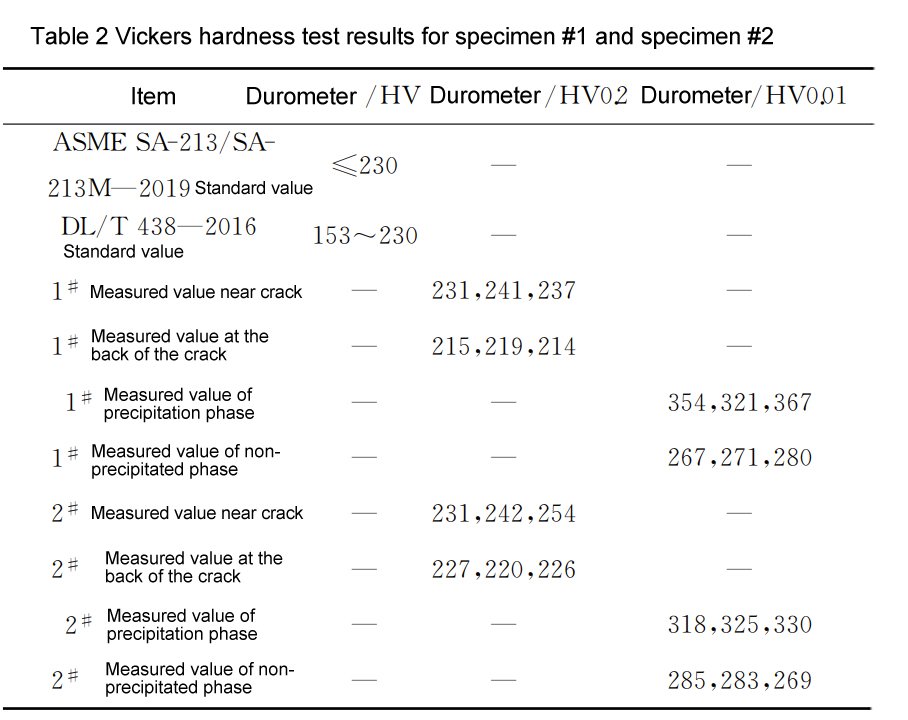
In accordance with ASME SA-213-2019 and DL/T438-2016, which are “Thermal Power Plant Metal Technology Supervision Regulations,” the established hardness requirement for S30432 steel pipes is not to exceed 230 HV. From the information in Table 2, it’s evident that the hardness on the inlet side and the outlet side near the crack slightly exceeds the upper limit of the standard. Conversely, the hardness at the back of the crack is slightly lower than the upper limit. Importantly, the hardness near the crack on the inlet and outlet sides is higher than the hardness at the back of the crack, and the hardness at corresponding positions on the inlet and outlet sides is quite comparable. Notably, the hardness of the precipitated phase on the inlet side and the non-precipitated phase is substantially higher than that of the non-precipitated phase.
These test results provide valuable insights into the composition and hardness characteristics of the burst pipe, helping us in our investigation.
1.4 Metallographic Examination:
We employed optical microscopy to observe different locations on the steam side of the 2# specimens and the burst 3# specimens. The cross-section micro-morphology of the 3# specimen is presented in Figure 3.
Figure 3 displays the polished state of the specimen’s morphology. On the crack surface, the opening is wider, and the tip is narrower, taking on a meandering shape with several branching patterns. There is also evidence of oxidation around the crack area. Inside the sample, we observed numerous holes and microcracks, primarily situated along grain boundaries. Additionally, we noted a significant number of white, bright-colored massive or elongated precipitation phases. These phases were distributed along the grain boundaries in the form of strings. Importantly, the holes and microcracks were present around these massive precipitation phases and extended along the grain boundaries. Notably, the internal microcracks had not yet connected with the cracks on the outer surface.
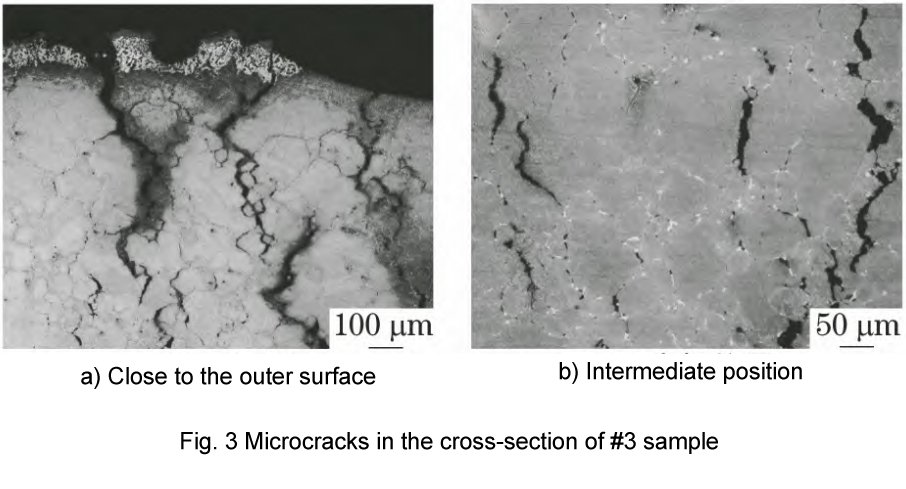
We also conducted a metallographic examination of different positions on the 1# specimens, with the results shown in Figure 4. In these examinations, we observed a layer of oxide skin at the fracture, along with precipitation phase coarsening and string-like connections to grain boundaries in the vicinity of the burst. This indicates aging in these areas.
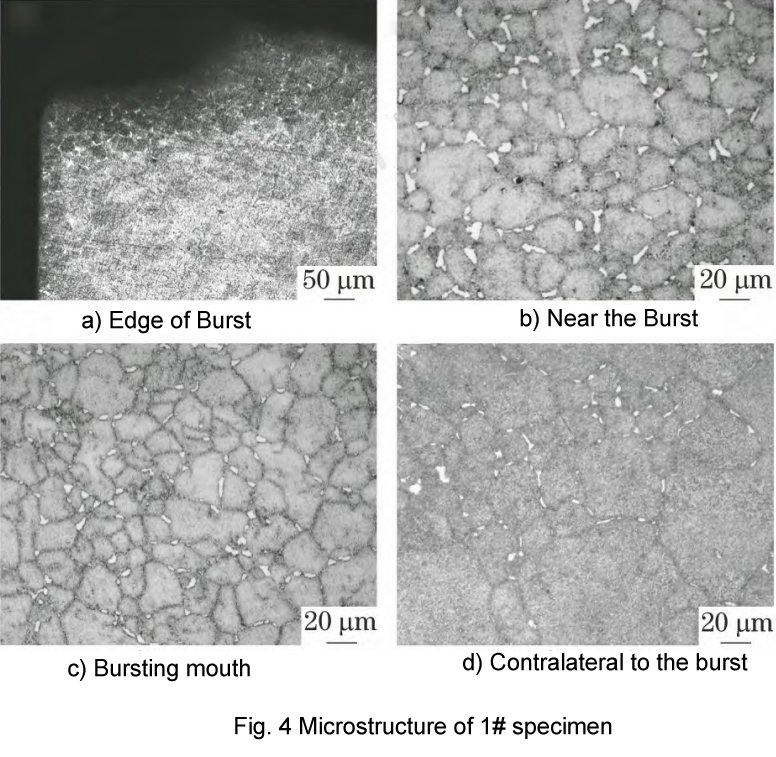
Based on the DL/T1422-2015 standard, the aging level near the burst location was rated at 5, signifying complete aging. The burst’s side had an aging level of 4.5, indicating heavy aging.
Notably, the 1# samples exhibited white-colored block or long precipitation phases distributed along the grain boundaries, much like the 3# samples. These white-colored block precipitates were most prominent near the burst mouth, gradually diminishing in size, length, and quantity as they extended along the circumference.
The common precipitation phases found in austenitic stainless steel include carbonitrides like M23C6, MX, and Z phases, as well as intermetallic compounds such as σ phase and Laves phase. In conventional 304H steel, the σ-phase and Laves phase typically appear only after prolonged exposure to high temperatures.
In the case of the burst pipe, the bright white precipitation phases at the grain boundaries were initially inferred to be σ phases based on their morphology, distribution, and color. The average length of these σ phases was approximately 4 μm, but they notably grew significantly in the vicinity of the burst, with an average length of about 8 μm. The presence of holes around these bright white precipitation phases was also observed.
These findings shed light on the microstructural characteristics of the burst pipe and the potential role of σ phases in the failure.
Our analysis extended to scanning electron microscopy (SEM) and spectral examination, which revealed additional details about the composition and fracture modes of the specimens.
1.5 SEM and Spectral Analysis:
We placed the 1# sample under SEM observation, and the results are depicted in Figure 5. The examination unveiled the presence of numerous blocky and elongated precipitation phases along grain boundaries, as well as the diffuse precipitation of granular second phases within the grain. Furthermore, there were observable holes surrounding the larger precipitation phases. Notably, the individual blocky precipitation phase boundaries exhibited intermittent microcracks.
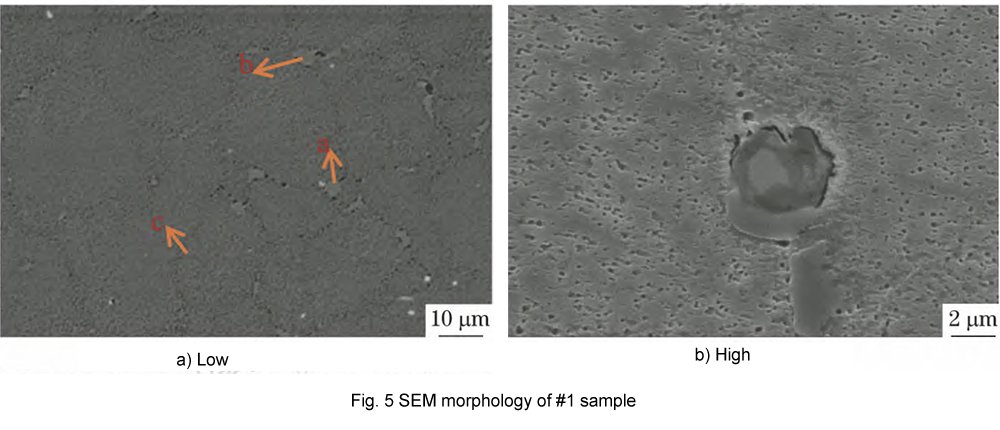
Figure 5 showcases the spectral analysis of the bulk precipitation phases. The results are provided in Table 3. The analysis indicated that the phase found at the grain boundaries of the burst pipe samples was consistent with Fe-Cr phase, presumably σ-phase, and a Cu-rich phase. These findings align with our earlier metallographic examination and microhardness test results.

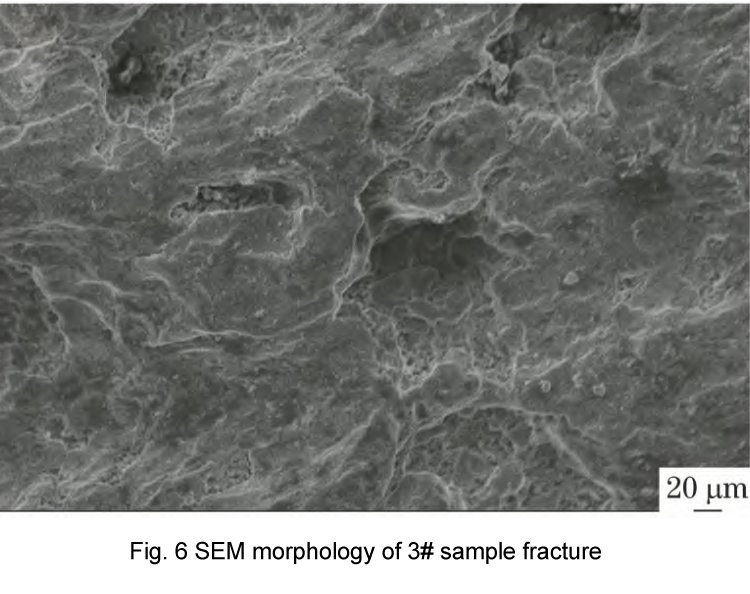
The 3# sample was also subjected to SEM analysis, and the morphology of the sample’s fracture is presented in Figure 6. The fracture surface displayed a layer of oxide skin, a rough and granular section, and notably, no evidence of tough fossa. This suggests that the fracture occurred in a brittle mode.
These SEM observations and spectral analyses provide crucial information about the microstructural features and fracture mode of the specimens, contributing to our understanding of the burst pipe’s condition and potential failure mechanisms.
In our comprehensive analysis of the S30432 steel and the failure of the burst pipe, several key findings have emerged:
- Microstructural Changes: The normal structure of S30432 steel consists of austenite with a small amount of a secondary phase. However, in the examined 1# and 3# samples, we observed white, bright, large, or long precipitates along the grain boundaries. These precipitates were significantly coarser than what’s typically seen in the normal microstructure. Energy spectrum analysis confirmed that these white-colored precipitates were Fe-Cr rich σ phases.
- Hardness Variations: The microhardness of the σ-phase was notably higher than that of the intracrystalline dispersed precipitates. Additionally, the hardness of the specimen matrix was close to the upper limit of the standard. The σ-phase is a common intermetallic compound with a tetragonal crystal structure, and its precipitation restricts dislocation slip during deformation, resulting in increased hardness.
- Precipitation Patterns: The σ phase primarily precipitates along grain boundaries or twin grain boundaries. Notably, the σ phase’s coarsening is most significant at trident grain boundaries. The presence of the σ phase was observed at various locations in the ring on the steam side of the vent. The average length of the bright white precipitation phase (σ phase) on the side opposite the vent was about 4 μm, while the σ phase near the vent had grown significantly, with an average length of about 8 μm. This suggests that higher temperatures are conducive to the precipitation of the σ phase. In fact, the analysis indicates that the actual operating temperature of the burst pipe section likely exceeded 750 °C.
- Failure Mechanism: The burst pipe’s rupture occurred along the grain boundaries, where coarsening of the σ-phase precipitates was observed. These coarser precipitates were often accompanied by the formation of holes and cracks. As the cracks developed to a certain extent, the persistent plasticity and strength of S30432 steel significantly decreased. Ultimately, under the influence of the internal pressure within the pipe, it burst.
In summary, the presence and coarsening of the σ phase, likely due to operating temperatures exceeding 750 °C, played a crucial role in the failure of the burst pipe. This phase promoted the formation of cracks and weakened the material’s mechanical properties, leading to the ultimate rupture of the pipe.
In conclusion, our analysis of the burst pipe from the screen-type superheater has led to several key findings:
- High-Temperature Operation: The burst pipe, particularly near the mouth of the rupture, exhibited a significant number of cracks and holes, along with elevated hardness and aging characteristics. Notably, there was a pronounced precipitation of large or long σ-phase along the grain boundaries. The closer the burst was to this location, the more abundant and coarser the σ-phase became. This strongly suggests that the burst pipe section had been operating at temperatures exceeding 750 °C for a considerable period.
- Temperature-Dependent Precipitation: The burst pipe’s annular specimens displayed the presence of σ-phase throughout the entire circle. The size of the σ-phase near the burst location was notably larger than on the opposite side, with an average length of about 8 μm. This observation reinforces the notion that higher temperatures are more conducive to the precipitation and coarsening of the σ-phase.
- Role of σ Phase: The presence of holes and micro-cracks around the σ-phase indicates that, due to overheating, the σ-phase precipitated and coarsened within the screen superheater. This, in turn, led to the formation of cracks, ultimately resulting in the rupture of the pipe.
In summary, the burst pipe’s failure can be attributed to the precipitation and coarsening of the σ-phase, which is strongly linked to high-temperature operation. The formation of cracks initiated around the σ-phase, ultimately leading to the catastrophic failure of the pipe. Understanding these factors is crucial for addressing and preventing similar incidents in the future.
[Source] Meng Dianwu, Yin Ying, Zhang Xuexing, Gao Dawei,Cause of tube explosion of platen Superheater in ultra-supercritical unit
DHB Boiler
Discover The Superior Quality And Cutting-Edge Technology Of DHB Boilers. Explore Our Range Of Biomass Boilers, Waste Heat Boilers, And More. Take Your Industrial Operations To New Heights With DHB Boiler.