Among various accidents in boiler and auxiliary equipment, boiler damage and tube bursting are the most common, especially in coal-fired power plants where boiler tube bursting is a prevalent occurrence, accounting for around 70% of overall incidents. Within the category of tube bursting, water-cooled wall failures make up a significant 80%. Once tube bursting occurs, a large amount of steam-water mixture is expelled, disrupting the stable operating conditions of the boiler, causing violent fluctuations in water level, and impacting the combustion environment. In severe cases, it may even lead to boiler extinguishment. Most tube bursting incidents require a shutdown for resolution, causing unplanned downtime for the enterprise. The losses incurred with each unplanned shutdown range in the tens of thousands to millions of yuan, and in severe cases, it may pose a threat to personal safety. Therefore, giving due attention to boiler tube bursting incidents is a crucial aspect of safety management in factories and power plants. In response to this issue, power plants must prioritize a thorough analysis of the causes and actively research and implement effective control measures to ensure the safe operation of boilers.
1. Boiler Tube Bursting Failures
Boiler tube incidents primarily involve the bursting of four tubes: economizers, water-cooled walls, superheaters, and reheaters. In a certain power plant in Shanxi, there are 2X600 MW coal-fired generating units with the model SG-2027/25.4 M970. The boilers operate with a supercritical parameter, variable pressure, spiral-coil direct-flow design. According to statistics, among all the leakage incidents in this power plant’s boilers, water-cooled wall leakage accounts for approximately 80% of all leakage incidents. This issue poses a serious challenge to the safe production of the power plant and the normal operation of the boilers. To reduce the occurrence rate of water-cooled wall leakage incidents and ensure the safe and stable operation of the power plant, it is necessary to analyze the causes of water-cooled wall leakage from multiple perspectives. The following analysis focuses on the common causes of boiler water-cooled wall leakage to effectively guarantee the power plant’s safety and stability.
2. Main Causes of Tube Bursting
2.1 Poor Boiler Feedwater Quality Leading to Scaling and Overheating Explosion of Water-Cooled Wall Tubes
Water with a high mineral content, such as calcium (Ca) and magnesium (Mg) salts, is referred to as “hard water.” Water that has had most or all of its calcium and magnesium ions removed is called softened water. The steam boiler typically requires an outside water hardness of less than or equal to 0.03 mmol/L. As the feedwater evaporates multiple times in the boiler, calcium bicarbonate (Ca(HCO3)2) and magnesium bicarbonate (Mg(HCO3)2) originally dissolved in the water decompose in boiling water, releasing carbon dioxide (CO2) and forming insoluble calcium carbonate (CaCO3) and magnesium hydroxide (Mg(OH)2), sometimes also generating MgCO3. The chemical reactions are as follows:
Ca(HCO3)2 = CaCO3 (precipitate) + CO2↑ + H2O
MgCO3 + H2O = Mg(OH)2 (precipitate) + CO2↑
Over time, as the concentration of these poorly soluble salts reaches the saturation limit at a certain temperature, insoluble compounds, primarily composed of calcium and magnesium ions, precipitate out of the water, forming flocculent white impurities on the inner walls of the water-cooled wall tubes. As the impurity concentration in the water-cooled wall increases, white solid precipitates (generally crystals) form in the water solution. If the precipitate does not adhere to the heated surface and remains suspended in the water, it is referred to as sludge or sediment. If the crystallization process of the precipitate occurs on the heated surface and the water contains impurities such as carbonates, colloids, bacteria, and organic matter, carbonate acts like sand and stones in concrete, while colloids, bacteria, and organic matter act like cement. When colloids, bacteria, organic matter, and carbonate work together, hard scale is formed under high-temperature conditions, firmly bonding to the heated surface, known as scaling.
Scaling typically adheres to the inner surface of water-cooled wall pipes. In the presence of scale, the thermal conductivity is significantly lower than that of steel, often differing by two orders of magnitude. Even if the scale thickness is small, it affects the metal’s heat transfer, and if the metal does not cool down in time, the temperature will exceed the allowable working temperature, leading to overheating and tube bursting. Furthermore, scale acts as a barrier between the steel pipe and the steam-water mixture. The intense flames in the furnace heat the steel pipe to a red-hot state, reducing the strength of the steel pipe. If cracks appear in the scale, water immediately leaks onto the hot steel pipe, rapidly evaporating and causing a sharp increase in pressure inside the boiler tube. This can result in water-cooled wall tube bulging or tube bursting accidents. Poorly conducting scale reduces the heat transfer effectiveness on the heated surface, causing the heat released from fuel combustion to be inefficiently transferred to the feedwater. This deterioration in heat transfer conditions not only leads to an increase in flue gas temperature and higher flue gas heat losses, reducing boiler thermal efficiency, but also, due to thermal expansion and contraction and uneven stress, can cause damage to the heated surface of the boiler, greatly increasing the risk of boiler rupture and even explosion.
2.2 Wear and Thinning of Water-Cooled Wall, Reduced Wall Thickness, and Tube Bursting
Wear often occurs at water-cooled wall tubes such as the burner outlet, furnace outlet, and observation holes. The wear condition of the water-cooled wall in Boiler #4 at a power plant in Shanxi is presented in Table 1.
Table 1: Inspection Records of Water-Cooled Wall Tube Wall Thickness in Boiler #4 at a Power Plant in Shanxi
Inspection Point | Design Thickness (mm) | Thickness After Wear (mm) | Thinning Rate (%) | Pass/Fail |
---|---|---|---|---|
1 | 5.5 | 3.3 | 40 | No |
2 | 5.5 | 3.7 | 32.7 | Yes |
3 | 5.5 | 3.9 | 29.1 | Yes |
4 | 5.5 | 3.4 | 38 | No |
The main causes of boiler tube wear are fluid scouring due to ash particles, coal powder airflow, leakage, or improper operation of soot blowers, and straight-flow burners causing deflection. The analysis of the causes of water-cooled wall tube wear is as follows:
- The coal powder used in the boiler combustion has a low quality, and the particle size is too large, resulting in high kinetic energy. The rapid flow of coal powder particles, incompletely burned particles, and ash causes severe scouring of the water-cooled wall, leading to the peeling of a small amount of metal from the outer surface of the metal pipe and causing wall thinning. The higher the coal ash concentration and flow velocity, the more severe the wear on the heated surface, eventually leading to water pipe leaks.
- Some power plants have boilers with structural issues due to non-compliance with regulations and related requirements during assembly. Improper welding joints, overlapping seams, and uneven surfaces during installation can result in a rough wall surface, easily causing severe wear on the water-cooled wall tubes during operation. While water-cooled wall tubes in circulating fluidized bed boilers generally experience wear, the annual wear rate is relatively small, indicating uniform wear.
- Another cause of wear is the improper installation or damage of burners, resulting in wear on the tube walls around the burners. In a primary air-coal powder mixture, the coal powder content is approximately 200-700 g/kg of air. When the primary air is injected into the furnace, improper burner installation angles or improper soot blowing can cause high-speed scouring of the water-cooled wall, leading to wear and thinning. In a water-cooled wall leakage incident that occurred at the power plant in Shanxi in June 2017 (as shown in Figure 1, displaying the cut section of the leaking pipe), it is evident that the wear on the water-cooled wall tube wall was quite severe, resulting in thinning and subsequent leakage.
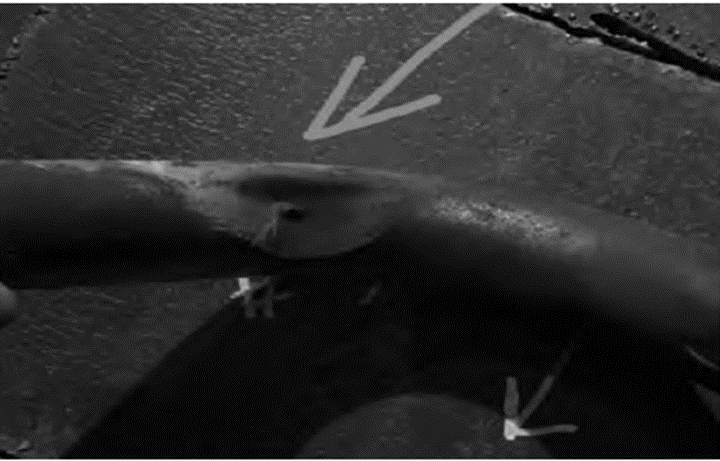
Figure 1: Wear on the Water-Cooled Wall in Boiler #4 at a Power Plant in Shanxi
2.3 Abnormal Steam-Water Circulation Leading to Overheating and Tube Bursting
When the flow direction or velocity of the steam-water mixture on the heating surface of the evaporator does not follow the designed flow, resulting in stagnation, backflow, or the formation of a free water level, it is termed as abnormal water circulation. This leads to improper distribution of steam and water, causing the water film on the evaporative heating surface to be disrupted. This disruption prevents this portion of the metal from receiving timely cooling, resulting in the metal temperature exceeding the permissible working temperature.
Overheating induces changes in the metal structure of the tubes, weakening their mechanical properties. The tube’s ability to resist the pressure of the working medium inside (strength) decreases as the temperature rises. Under internal pressure, the tube undergoes plastic deformation, blistering, swelling, and bursting, ultimately causing tube wall leakage, as depicted in Figure 2.

Figure 2: Tube Bursting in Boiler #4 at a Power Plant in Shanxi
2.5 Water-Cooled Wall Corrosion
2.5.1 Corrosion Beneath Scale Inside the Tube
The inner surface of water-cooled wall tubes is covered with a protective layer of Fe3O4. This protective film is most stable when the pH of the boiler water is between 9 and 10, resulting in minimal corrosion inside the tube. If the pH of the boiler water exceeds the standard, the protective film in the furnace tube is damaged. The concentration of NaOH required decreases as the temperature increases, and the reactions are as follows:
Fe3O4+4NaOH=2NaFeO2+Na2FeO2+2H2O
Fe+2NaOH=Na2FeO2+H2
At high temperatures, NaOH can also react with carbon to produce methane (CH4):
Fe3C+6NaOH→3Na2FeO2+CH4+H
During this corrosion process, CH4 and H2 accumulate in the microgaps at the grain boundaries. As the reaction continues, the accumulation of methane at the grain boundaries increases, leading to the expansion of the intergranular space. Since the molecular size of methane is larger compared to the volume occupied by hydrogen atoms, methane cannot diffuse within the steel. This creates internal pressure within the metal, forcing the expansion and separation of grain boundaries, causing intergranular cracks. Ultimately, at high temperatures, this process can lead to tube bursting.
2.5.2 High-Temperature Corrosion Outside the Tube
At elevated temperatures, sulfur elements participate in various corrosion processes on the outer surface of the tube. Low-quality coal with a sulfur content greater than 1.2% tends to release reducing gases such as CO and H2, as well as corrosive gas H2S during combustion. The released sulfur reacts with the metal, leading to the formation of FeS (iron sulfide), causing high-temperature corrosion of the tube wall. The temperature of the water-cooled wall tubes on the flue gas side is typically around 420°C, falling within the temperature range where intense high-temperature corrosion of the metal occurs.
3. Effective Measures to Prevent Boiler Water-Cooled Wall Leaks
The analysis of the causes of boiler water-cooled wall leaks emphasizes the importance of addressing leakage issues. Different methods must be implemented based on the specific causes of the leaks.
3.1 Measures for Scaling Treatment of Water-Cooled Walls
3.1.1 Boiling and Acid Cleaning
Boiling and acid cleaning not only remove rust and oil deposits generated during the manufacturing, transportation, and installation of boilers but also quickly bring the water vapor quality during the initial ignition to the required standard.
3.1.2 Improving Feedwater Quality
Strict measures should be taken to ensure the boiler water quality, reducing water hardness to meet the requirements of industrial boiler water quality GB1576.
(1) Ion Exchange Method
Sodium ion exchange resin is used to replace calcium and magnesium ions in water, reducing hardness to soft water.
(2) Reverse Osmosis Method
This process uses pressure higher than osmotic pressure to extract fresh water from a solution through a selectively permeable membrane that allows only water, not solutes, to pass. Reverse osmosis is also known as RO.
(3) In-Furnace Chemical Treatment
Trisodium phosphate, an effective inhibitor of scale formation, is a white to colorless crystal. Adding trisodium phosphate to the furnace breaks down into disodium hydrogen phosphate and sodium hydroxide in the solution. It reacts with calcium and magnesium ions in the boiler water to form a soft and precipitated phosphate scale. The scale settles in the water-cooled wall header, and regular draining removes it from the furnace.
Water softening can only reduce hardness, not completely remove salts. In areas with poor water quality, low-salt desalinated water should be used to effectively reduce boiler feedwater hardness. Additionally, the hardness of the condensed water returning to the boiler from the production process must not exceed the specified value. Improving feedwater quality reduces the blowdown rate, minimizing energy and heat losses, and extending the boiler’s lifespan.
(4) Sampling and Testing of Boiler Water and Steam Systems
To prevent issues such as salt accumulation, scaling, and corrosion in the boiler’s internal pipes, water and steam quality should meet the standards of industrial boiler water quality GB1576. Therefore, regular sampling and testing of boiler water and steam are necessary, and corrective actions should be taken promptly if results are unsatisfactory.
(5) Continuous Blowdown
As boiler water evaporates, the salt concentration increases, surpassing that of feedwater. To maintain good steam quality, continuous blowdown at the saltiest part of the boiler water helps rapidly improve water and steam quality.
(6) Periodic Blowdown
When water treatment equipment malfunctions or is incomplete, the feedwater to the boiler becomes turbid, with increasing impurities like sand, mud, and residues of calcium and magnesium ions. Failure to drain these impurities may lead to poor cooling and the risk of tube blockages. Therefore, periodic blowdown at the water-cooled wall header is essential. Boiler operation generally involves a scheduled blowdown every 8 hours, expelling impurities such as mud and water residues from the boiler water to swiftly adjust water quality and prevent tube blockages.
3.1.3 Ensuring Tightness of Steam Equipment and Steam-Water Pipelines
Leakage in steam equipment and steam-water pipelines can introduce cooling water or impurities into condensed steam, causing an excess of calcium and magnesium salts. This is a significant factor contributing to scale formation. Therefore, when an increase in hardness is detected in the steam equipment or boiler feedwater, it is crucial to promptly inspect and eliminate any leaks in steam equipment and steam-water pipelines.
3.2 Prevention and Control of Wear and Leakage
Preventing wear on water-cooled wall tubes primarily involves improving the quality of coal powder. The particle size distribution of coal powder (R90) should be controlled between 10% and 13% to avoid excessive particle size. During operation, it is essential to promptly clear slag near the burner nozzle to prevent buildup.
Additionally, a common practice is to affix welded short steel bars or install anti-wear tiles on easily worn areas of the pipes. Due to the good contact between the short steel bars and the water-cooled wall, effective cooling is achieved, making them less prone to damage. In a power plant in Shanxi, due to recurrent incidents of wear and leakage in water-cooled walls, a layer of anti-wear tiles was added at the bend where wear was prominent, preventing the direct impact of high-speed ash and air mixture on the pipe wall, with positive results.
3.3 Prevention and Control of Over-Temperature Leakage
To prevent over-temperature incidents leading to tube bursting due to cycling faults, uniform fuel supply, stable combustion, a well-established air dynamic field inside the furnace, and even distribution of air and coal powder are necessary. Adjusting the position of the flame center during operation helps avoid wall-attached combustion. Proper use of burners should be ensured to prevent excessively high temperatures in the burner area. Boiler operating load should be neither too high nor too low to prevent overheating of heat surfaces. To prevent heat transfer deterioration, the design should consider factors such as not oversized water-cooled wall tube diameter, a reasonable number of parallel tubes in the water-cooled wall tube assembly, rational tube grouping, and appropriately increased cross-sectional area of downcomers. Alternatively, methods such as using internally threaded tubes (as shown in Figure 3) can be employed:
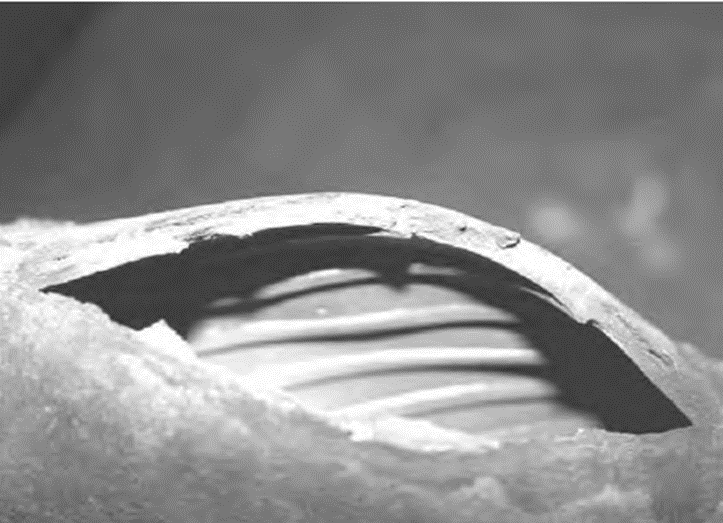
Fig. 3 Threaded pipe in the water-cooling wall pipe
3.4 Preventive Measures for Poor Welding Quality in Furnace Tubes
(1) Use small bevel angles within the permissible range of the welding procedure as much as possible.
(2) Perform preheating work to raise the temperature appropriately before the formal start of spot welding.
(3) Use double-person symmetric alternate welding. Alternately and evenly welding with two people provides a certain level of temperature assurance between various layers of the weld, effectively reduces welding stress, and minimizes deformation after welding completion.
(4) Ensure that gaps, including the joints between tube rows, match sealing, and the gaps between tube rows, are less than 4 mm, meeting the explicit requirements specified in relevant regulations.
(5) After completing welding work, conduct 100% radiographic testing. With a qualification rate reaching 95%, any non-compliance requires re-welding. Simultaneously, effective control is applied to the overall installation dimensions of the tube row.
3.5 Prevention and Control of Corrosion Leakage
To prevent corrosion under scale on water-cooled wall tubes, it is essential to enhance feedwater quality, conduct thorough chemical testing, and minimize impurities and NaOH content in feedwater. To prevent external corrosion of water-cooled walls, proper installation of burner angles, improvement of combustion environments, avoidance of direct flame impingement on water-cooled walls, appropriate increase in excess air ratio, rational air distribution, and adjustment of combustion to reduce the production of reducing gases near the water-cooled wall are recommended. Additionally, increasing the flow velocity of steam-water inside the tubes helps prevent excessive temperatures and intensifies corrosion. Other preventive measures include using high-alloy steel, permeable aluminum tubes, or applying silicon carbide coatings.
4 Conclusion
Boilers serve as the core equipment in power plants and enterprises, directly impacting their economic and environmental performance. The causes of boiler tube rupture are diverse, often resulting from the combined influence of various factors. Therefore, preventing boiler tube ruptures requires comprehensive measures, including installation, operational management, and inspection. Additionally, employing high-fidelity 3D models of boilers facilitates the correlation of three-dimensional representations with operational data, aiding in the real-time monitoring of boiler conditions.
A real-time monitoring system, utilizing historical data from the unit, helps track the occurrence time and development trends of leaks. Analyzing the creep expansion trends and residual life of the boiler’s heating surfaces provides valuable insights for developing maintenance plans. This approach offers crucial guidance for focused inspections, effectively preventing, eliminating, or minimizing incidents such as over-temperature ruptures and leaks in water-cooled wall tubes. Implementing these measures ensures the overall safety, reliability, and longevity of boiler systems, safeguarding both economic and environmental interests for power plants and enterprises.
DHB Boiler
Discover The Superior Quality And Cutting-Edge Technology Of DHB Boilers. Explore Our Range Of Biomass Boilers, Waste Heat Boilers, And More. Take Your Industrial Operations To New Heights With DHB Boiler.