Circulating Fluidized Bed Boiler (referred to as CFB boiler) technology represents a cutting-edge, high-efficiency, and environmentally friendly combustion method. It has gained widespread recognition and adoption both domestically and internationally. However, a significant challenge associated with this technology is the wear and tear experienced by the water-cooled walls within the boiler. If not addressed appropriately or if improper measures are taken, this issue can exacerbate and lead to severe deterioration.
Statistics reveal that over 50% of CFB boiler shutdown incidents are attributed to wear and tear-related problems. This issue is particularly pronounced in northern regions where the utilization of low-calorific and poor-quality coal is prevalent. Such conditions result in frequent occurrences of abrupt shutdowns, posing a substantial challenge for boiler units. Consequently, various anti-wear technologies have emerged and continue to evolve to combat this predicament.
Our company has deployed five sets of CFB boiler, each with rated evaporation capacities of 75 t/h, 130 t/h, and 180 t/h, respectively. These boilers operate at a medium temperature and medium pressure of 3.82 MPa/450 ℃. Additionally, cogeneration systems are integrated into three of these sets, featuring 15 MW steam turbine generators. The primary objective of employing these boilers is to ensure a consistent and reliable steam heat source to support chemical industry production processes.
The service life of these domestic boilers extends beyond a decade, demonstrating their robustness and durability. It’s important to note that variations in furnace designs and manufacturing origins contribute to differences in wear patterns. Addressing wear protection amid these disparities presents a formidable challenge. Through practical experience, we have successfully introduced the active inflow anti-wear technology, coupled with effective follow-up measures. As a result, the issues pertaining to wear on the boiler’s water-cooled walls have been effectively mitigated and resolved.
1. Mechanism of Water-Cooled Wall Wear
The term “boiler heating surface” primarily encompasses the surfaces within the CFB boiler steam system shell and the combustion system, where high-temperature flue gases interact with metal surfaces, enabling efficient heat transfer. This paper’s specific focus centers on examining wear phenomena encountered by the heat transfer surfaces of the CFB boiler furnace chamber, commonly referred to as water-cooled walls. In the context of this study, water-cooled wall wear pertains to the wear experienced by these crucial heat transfer surfaces. Despite the original design’s intention to shield the CFB boiler’s water-cooled walls from wear, with dense phase regions protected by castings and transition zones featuring water-cooled walls, practical experience demonstrates that wear remains a persistent challenge within the CFB boiler environment.
Several factors contribute to the wear of water-cooled walls, including the inherent structural characteristics, coal particle size, furnace temperature, load rate, differential pressure, ash concentration, oxygen content, and other variables. It’s crucial to recognize that each boiler’s wear condition varies due to differences in coal quality, operational practices, and other operational factors.
1.1 Categories of Wear
Wear experienced by the furnace water-cooled walls can be categorized into four distinct types:
- Impact and Cutting Wear: This type of wear occurs as flue gas particles impact the heating surface, causing cutting and abrasion.
- Flow Wear: Material particles move along the heating surface, generating wear through contact with the surface.
- Direct Scouring Abrasion: Local jets of bed material create abrasive forces on adjacent surfaces, leading to direct scouring.
- Irregular Geometric Shape Abrasion: Irregularities in the geometric shape of surfaces result in local eddies, leading to abrasion.
1.2 Modes of Wear
The predominant mode of wear observed on most boiler water-cooled wall heating surfaces is material wear. Solid particles with specific velocities and angles collide with the surface, inducing erosive wear. When particles impact the surface in parallel or at shallow angles, it’s referred to as scouring wear. Conversely, impact wear occurs when particles collide at steeper angles, leading to surface deformation and detachment. These two fundamental modes, scouring and impact, constitute the basis of wear mechanisms. When examined under a microscopic lens, these modes can further be subdivided into four distinct forms, as depicted in Figure 1.

1.3 Principal Areas of Wear and Deterioration
The wear and deterioration of the water-cooled walls in CFB boiler primarily concentrate within three distinct regions within the furnace chamber. These areas, significant both in terms of wear occurrence and severity, warrant focused attention for wear prevention and control measures.
(1) Transition Zone: This area lies between the castable lining and the water-cooled wall. It encompasses the upper portion of the tube bundle, situated above 0.5 meters in height. Typically occurring around elbows, this space is characterized by a mixture of gas and solid phases, resulting in a high concentration of material particles. The regional height facilitates fluidization, often in the form of bubble or turbulent states. Material surges downstream along the wall, accompanied by upward movements and local vortex formations. This dynamic environment intensifies the interaction between heated surfaces and particles, fostering pronounced scouring and impact wear. The distinctive inverted “eight” wear patterns evident on the tube bundles highlight the severity of wear in this region.
(2) Furnace Chamber Corners and Exit Region: Within the corners of the furnace chamber, the accumulation of fluidized media and the presence of unsteady airflow give rise to wear-intensive conditions. Vortex formations are prone to occur in this context, exacerbating wall flow instability and consequent wear. In the furnace exit region, wear results from the abrupt steering of airflow, leading to high-concentration two-phase flow and the centrifugal expulsion of ash particles onto the water-cooled walls. The typical wear pattern in this area manifests as impact wear.
(3) Irregular Pipe Wall Zone: This category encompasses various irregularities within the pipe walls, including wall penetrations, bends, weld seams, and instrumentation elements. Components such as manhole doors and fire viewing holes are also susceptible to wear. Localized wear in these regions stems from flow disruptions caused by irregularities, creating vortex formations. Even minor geometric irregularities, when subjected to the cyclical effects of scrubbing by vortices, can result in significant localized wear.
Careful attention and intervention in these identified wear-prone regions are pivotal to effectively addressing and mitigating the wear and tear experienced by water-cooled walls.
2.Suitability of Active Infusion Technology for CFB Boiler
Since 2012, our strategy to combat water-cooled wall abrasion in CFB boilers has predominantly revolved around the utilization of metal anti-friction spraying techniques. These measures have been strategically focused on safeguarding critical wear-prone areas, driven by the primary objective of offering precise and effective protection. As an integral part of this approach, we diligently schedule furnace inspections at approximately four-month intervals on average. Despite these proactive efforts, the recurring issue of one to three water-cooled wall pipe bursts and the resulting furnace shutdowns annually still persists, posing a substantial and ongoing hazard to chemical production operations within the CFB boiler environment.
To enhance the durability of the protective coatings, we explored the possibility of incorporating ultra-wear-resistant spray materials, including liquid alloys, wear-resistant ceramics, and cladding fusion. However, it should be noted that while these advanced spray materials differ from traditional thermal spraying, they fundamentally extend the wear-resistant cycle. Yet, they remain unable to fully counteract the rapid localized scouring experienced at measurement points, corners, and other irregularities. Consequently, the inherent risk of increased probability for leakage remains unmitigated.
In light of these limitations, the need for an alternative and more effective solution becomes evident in order to comprehensively address the persistent wear-related challenges faced in our operations.
2.1 Active Infusion Technology
Active infusion technology involves the deployment of a well-structured grid composed of large protective nets that envelop the vegetation’s surface. This grid effectively blocks the airflows that might otherwise attack the surface layer of the vegetation. This infusion and shielding mechanism represent the practical implementation of active infusion technology. This technology, referred to interchangeably as the “warp and weft grille” or “grille protection” technology, encompasses the assembly of metal deflector plates into a mesh-like protective structure. These deflector plates, typically fashioned from CrNi-system austenitic heat-resistant steel castings, possess a thickness of 5 mm. Each deflector plate measures 300 mm in length, and these pieces are intermittently welded to secure the water-cooled wall fins. The cross plates within the grille are equipped with semi-circular holes to ensure they remain open and don’t come into direct contact with the water-cooled wall tubes. This design guarantees both the reliability of the welds and synchronization during thermal expansion, effectively resulting in the formation of a covered metal grille protection network over the water-cooled wall’s heat surface.
The underlying principle of warp and weft grille plate active infusion technology is grounded in its ability to impose active constraints on the gas-solid two-phase flow within the furnace chamber. By doing so, it progressively curtails the impact of wall flow on heated surfaces, thereby mitigating the scouring effect. This approach helps eliminate the localized irregular eddies that typically concentrate scouring, resulting in the concentration of wear. Consequently, the technology achieves its objective of directing flue gas in a manner that prevents material particles from causing abrasion on the heated surfaces through active guidance.
2.2 Implementation of Grid Protection
After extensive market research and comprehensive case studies, we adopted active infusion technology as the foundation of our protection measures. Collaborating with a proficient partner, we customized protection plans for each of the five CFB boiler, precisely addressing wear characteristics while minimizing disruption to capacity. For example, in July 2022, we installed grille mesh in the No. 5 furnace rated at 180 t/h, complemented by metal spraying for added protection.
Through thorough market consultation and comprehensive case studies, we integrated active infusion technology into our protection measures. Partnering with a technologically proficient company, we developed tailored protection plans for the five CFB boiler, minimizing capacity disruption while effectively addressing wear characteristics. As an illustration, in July 2022, we installed grille mesh in the 180 t/h-rated No. 5 furnace, accompanied by metal spraying for enhanced protection.
Following meticulous market research and extensive case studies, we adopted active infusion technology as the core of our protection measures. Collaborating with a proficient partner, we customized protection plans for each of the five CFB boilers, precisely addressing wear characteristics while minimizing disruption to capacity. For instance, in July 2022, grille mesh was installed in the No. 5 furnace rated at 180 t/h, complemented by metal spraying for added protection.
After thorough market consultation and comprehensive case studies, we embraced active infusion technology as the cornerstone of our protection measures. Collaborating with a technologically adept partner company, we developed distinct protection plans for the five CFB boiler, minimizing disruptions to capacity while effectively addressing wear characteristics. In the case of the No. 5 furnace rated at 180 t/h, grille mesh was installed in July 2022, alongside metal spraying in high-abrasion areas for added protection.
Extensive market consultation and in-depth case studies led us to adopt active infusion technology as the linchpin of our protection measures. Teaming up with a technologically proficient partner, we crafted custom protection plans for all five CFB boiler, expertly addressing wear characteristics while minimizing capacity disruptions. For instance, in July 2022, grille mesh installation in the 180 t/h-rated No. 5 furnace, coupled with metal spraying in high-abrasion zones, fortified our protective efforts.
Post three months of operational use, a furnace inspection revealed irregular abrasion marks in the transition area of the front and back walls. The previously applied sprayed layer also exhibited signs of abrasion. This prompted the implementation of compensatory measures, involving an increase in the number of tailored cross-plates. Subsequent furnace shutdowns, however, did not exhibit fresh signs of abrasion, and thickness measurements indicated negligible wall thinning. The robust performance of the grille network in mitigating wear and tear became evident during this period.
Comparing operational data extracted before and after the deflector plate installation showcased that the boilers operated at high load factors, with outputs reaching 90% (161 t/h). While minor fluctuations occurred in bed temperature, exhaust smoke temperature, furnace pressure difference, and induced draft fan current, these shifts were modest. Notably, the data indicated an increase in flue gas resistance and a reduction in exhaust heat loss, accompanied by a slight uptick in ash concentration within the circulation. Although the extracted data does not account for all operational variables and the constant nature of operating conditions, it is indicative of the technology’s successful integration in controlling wear and tear. The operational impact stemming from the introduction of active infusion technology has proven to be minimal, as demonstrated in Table 1.
Table 1 Parameters of the same load before and after installation of deflectors
Comparison unit | Evaporation (t/h) | Bed temperature (℃) | Furnace outlet temperature (℃) | Temperature of reclaimer (℃) | Exhaust temperature (℃) | Furnace Differential Pressure (Pa) | Induced draft fan current (A) |
Before the installation of the deflector | 161 | 920 | 862 | 908 | 128 | 549 | 42 |
After installation of the deflector | 161 | 910 | 922 | 931 | 136 | 523 | 36 |
2.3 Three Components of Wear
Practical observations have led to the generalization that the wear rate of the heated surface (expressed as wear per unit time) E (mm/106h) can be described using the following equation:
E=KpCpdp2U3
U – furnace flue gas particles of the flow rate, m / s;
dp – material particle diameter, mm;
Cp – flue gas particles concentration, kg / m3;
Kp – particle characteristics of the coefficient, generally take 10-3 of the outline unit.
Upon analysis of this expression, it becomes evident that a reduction in water-cooled wall wear primarily hinges on controlling the flue gas flow rate U. Theoretical considerations dictate that U should not surpass 6 m/s, with operational values often lying between 4.5 m/s and 5.5 m/s. The U value is interdependent on factors such as boiler size, load rate, primary and secondary air flows, and induced draft fan adjustments. Meanwhile, Cp, influenced by factors including coal feed internal circulation particles, external circulation ash superposition, and wind dynamics, concurrently impacts water-cooled wall wear and the furnace chamber’s thermal efficiency (η) and differential pressure (ΔP). When bed temperature and return material remain stable, advocating a higher Cp value is advisable. As for dp, it is a controllable parameter associated with coal feed particle size, serving as a critical determinant of thermal efficiency and wear.
3. Economic Aspects of Anti-Wear Measures
Having examined the compatibility of active infusion technology with circulating fluidized bed boiler (CFB boiler) applications earlier, we will now concisely delve into the economic significance and value of anti-wear measures, approaching this exploration from two unique angles.
Having explored the alignment of active infusion technology with circulating fluidized bed boiler (CFB boiler) applications in the preceding section, let’s now embark on a succinct journey to uncover the economic significance and value inherent in anti-wear measures. This investigation will unfold through the lens of two distinct perspectives.
Having delved into the synergy between active infusion technology and circulating fluidized bed boiler (CFB boiler) applications in the preceding segment, our focus now shifts to a concise exploration of the economic importance and value underpinning anti-wear measures. This dual-sided examination underscores the essence of our inquiry.
3.1 Crucial Role of Particle Size (dp)
Maintaining the stability of bed material boiling and bed temperature stands as fundamental prerequisites for the successful operation of CFB boiler. Amidst these requirements, the size of coal particles upon entry into the furnace—symbolized by dp—not only holds a pivotal role in wear and tear concerns but is also an indispensable factor influencing boiler combustion efficiency. Consequently, ensuring coal granularity as it enters the furnace emerges as a critical undertaking.
The recommended coal particle size typically ranges from 0 to 13 mm. Deviations from this range pose challenges: larger coal lumps can lead to bed deposition and the formation of stagnant zones, disrupting fluidization and ultimately causing coking and forced furnace shutdowns. Conversely, excessive fine particles can impair separator efficacy, potentially burdening the dust removal system at the back end and diminishing boiler efficiency. Carbon loss in fly ash due to high carbon elements further compounds the economic inefficiency.
In the case of our boilers, we utilize lignite with a calorific value of 15,960 to 18,900 kJ/kg. However, raw coal crushed for boiler use by tooth stick crushers results in uneven particle size, with particles ≥ 13 mm constituting over 14%. This poses threats to fluidization and wear resistance. Two years ago, we introduced a second screening and crushing stage to the coal conveyor belt, managing to control coal particle size within the 0 to 10 mm range.
The benefits of this adjustment were profound. Peak-period carbon loss due to ash burning saw a reduction of 2.6 percentage points compared to the original state, ultimately leading to an annual reduction of over 3,100 tons of standard coal consumption during low-load operations. Moreover, uniform particle size improved fluidization and wear resistance, leading to stable combustion, reduced bed temperature fluctuations, and improved furnace chamber pressure differences and return material stability.
Considering wear and tear, Figure 2 illustrates the recognized relationship curve between fixed flow rate dp and E. Altering coal particle size and utilizing parabolic point values, we found that reducing dp accelerates wear attenuation. Assuming coal particle size decreases from 15 mm to 10 mm, the relation E ∝ 2.25E remains consistent. Applying the formula E = Kp * Cp * dp2 * U3 with parameter averages of Cp = 3 kg/m3 and U = 5 m/s for the original dp of 15 mm, an annual wear value E corresponds to approximately 0.7 mm, projecting a water-cooled wall life of around 3 years considering a maximum thinning amount of 2 mm.
For coal particle size of 10 mm, assuming a 90% shielding efficiency due to grille plates, E corresponds to 0.031 (E years = 0.7/2.25 * 0.1). The introduction of active infusion technology and coal particle size alteration results in an annual water-cooled wall wear of 0.03 mm. While actual operation involves variable factors, this projection offers insight into the active infusion technology’s effectiveness in mitigating wear, while considering the three wear components to achieve near-complete shielding, thereby achieving high-efficiency anti-wear purposes.
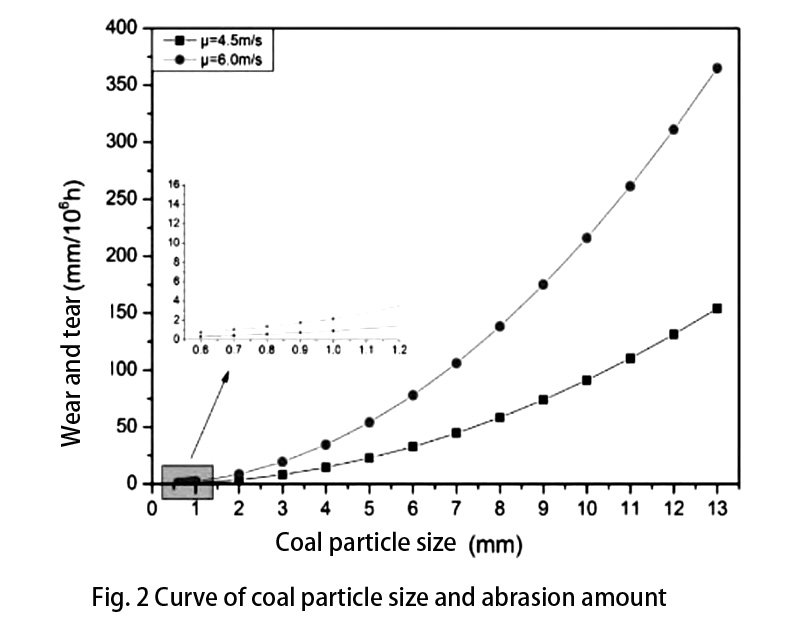
3.2 Comparison of Common Anti-Wear Technologies of CFB boiler
Leveraging insights from CFB boiler market feedback and extensive experience, we’ve meticulously curated an assortment of anti-wear technologies. Through a comprehensive analysis of key attributes related to the three prominently used anti-wear strategies—metal spraying, surfacing and welding, and active infusion—we unveil this comparison in Table 2. When evaluating the panorama of long-term economic considerations in the realm of CFB boilers, the pronounced edge of active infusion technology’s grille protection becomes even more apparent. This assertion is bolstered by the substantiating evidence within our specific CFB boiler case study.
Table 2 Comparison of the properties of three commonly used anti-wear measures
No. | Item | Metal spraying | Surfacing | Active infusion technology |
1 | Anti-friction method | Passive thickening anti-wear | Passive thickening anti-wear | Active protection against abrasion |
2 | Metal material | Commonly used materials are Cr series alloy powder, etc. | Wear-resistant alloy | Austenitic heat-resistant steel |
3 | Product performance | Hardness HRC 30-60, thickness of anti-wear layer 0.6-0.8 mm, tendency to brittle fracture | Hardness HRC45-60, thickness of anti-wear layer 1.5~4 mm | High temperature resistance, wear resistance, good weldability, no interference with the body of the pressure-bearing parts. |
4 | Construction cost | Differences in quality and cost, ranging from RMB 800~2000 per square meter | Higher cost; RMB 6,000~8,000 per square meter, 6~10 times higher than the price of spraying. | Cost-effective advantage, about 1,500 ~ 1,800 yuan per square meter |
6 | Operating life | Normal life of 1 to 2 years, high load transition area and wear-prone area protection effectiveness of 180 to 300 days | 2 years < life < 4 years, and the hardness of the fusion layer is very high, the subsequent maintenance is difficult. | Once installed, it can be used for 8 years, and subsequent maintenance is easier. |
7 | Warranty | 6 months to 1 year | 2 years | ≥3 years |
8 | Annual wear in the wear protection zone | >0.4 mm | 0.1~0.3 mm | <0.1 mm |
Conclusion
In view of the introduction of active infusion technology with metal grille plates and adjustments in coal particle size screening, three key findings emerge from safety and efficiency viewpoints within the context of circulating fluidized bed boilers (CFB boiler) :
(1) Practical Effect: Employing active infusion technology with metal grille plates, the approach centers on grille networks to combat wear components (Cp, dp, and U). This effectively achieves anti-wear goals, curbing pipe wall wear to less than 0.1 mm annually. Consequently, this innovative anti-wear technology garners substantial traction and adoption among numerous CFB boilers in China, driven by favorable market research results.
(2) Structural Characteristics: The grille protection network acts as a proactive anti-wear mechanism. Metal grille plates harmonize with pressure-bearing parts, sidestepping pitfalls associated with protective measures such as adhesion, fusion, and wall coverings. This mesh-like shield, devoid of inactive zones, safeguards against localized scouring and leaks. Designed to minimize smoke and wind resistance, the resilient, secure, and continuous heat production structure bolsters industry functions.
(3) Coal Particle Size: Tailored for a 200 t/h CFB boiler, an optimal coal particle size distribution spans 0.1 to 1 mm (40-50%) and 1 to 10 mm (remaining). This approach attains equilibrium in combustion, fluidization, circulation, slagging, and ash management. This equilibrium defines an economic sweet spot, governing energy consumption while enhancing water-cooled wall wear prevention and thermal efficiency. By striking this balance, operational safety and efficiency are elevated.
[Source] Li Qi-qi, Analysis on the Abrasion and Anti-Abrasion Economy of Water Wall of Circulating Fluidized Bed Boiler, Anhui Chemical Industry 2023[2]105-108
DHB Boiler
Discover The Superior Quality And Cutting-Edge Technology Of DHB Boilers. Explore Our Range Of Biomass Boilers, Waste Heat Boilers, And More. Take Your Industrial Operations To New Heights With DHB Boiler.